Guiding with an ONAG
The ONAG® uses a dichroic beam splitter (BS). The near infrared (NIR) light goes through the BS and it is used by your guider. Using NIR for guiding reduces significantly seeing effects making auto-guiding tracking better and smoother (see below for further information)
The starlight spectrum (power density versus wavelength) is given by the black body radiation theory. The spectrum of a black body, like a star, can be totally defined from its temperature (at equilibrium). It exhibits a quite heavy NIR tail which explains why most stars radiate a lot of energy in NIR.
Below there are the Hertzsprung–Russell main sequence star diagram showing the relationship between star temperature versus luminosity and a star spectral class table with the star relative proportions.
Available stars for NIR guiding are function of the temperature, luminosity, and their relative proportion in the main sequence and the milky way.
Typically when auto-guiding at f/10, or faster with few second exposure, there are many star to choose from.
main sequence diagram

Star spectral classes
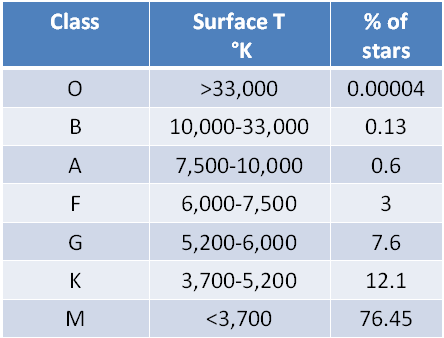
Now to understand how NIR works for guiding we need to take in account the guider sensor quantum efficiency (QE), the ONAG®’s optical transfer function, as well as the star radiation spectrum.
The overall system efficiency*, namely the ONAG® associated with a typical silicon sensor chip, can be found by the integration of the product between the chip QE and star spectrum for a given surface temperature, over the ONAG® bandwidth (~750nm to 1000nm),
The plot below shows the ONAG® visual magnitude offset, relative to a typical silicon sensor, against the guide star surface temperature (in °K).
When this offset is added to a given star visual magnitude the resulting value is the equivalent star visual magnitude which would provide the same sensor signal level using the full spectrum (no ONAG) than the ONAG resulting sensor signal (in NIR) for this star. The offset value is a function of the star surface temperature.
For instance a sun like star (surface temperature ~ 5800K, class G) of visual magnitude 10 when seen through the ONAG is equivalent at a sun like star of visual magnitude 10+1.33 = 11.33, without the ONAG, from a sensor signal level stand point.
Alternatively to reach the same sensor signal level in NIR (with the ONAG) one needs a 10-1.33 = 8.67 visual magnitude sun like star for an initial magnitude of 10 without the ONAG (full spectrum).
While a star with a surface temperature of 3700K (class M) and the same visual magnitude of 10 corresponds to a 0.76 magnitude offset.
In this case a star with the same surface temperature of a visual magnitude 10-0.76 = 9.24 provides the same sensor level with an ONAG (NIR).
* Atmospheric extinction and optics efficiency are neglected since they are not dominant here.
ONAG visual magnitude offset versus guide star surface temperature (silicon sensor)
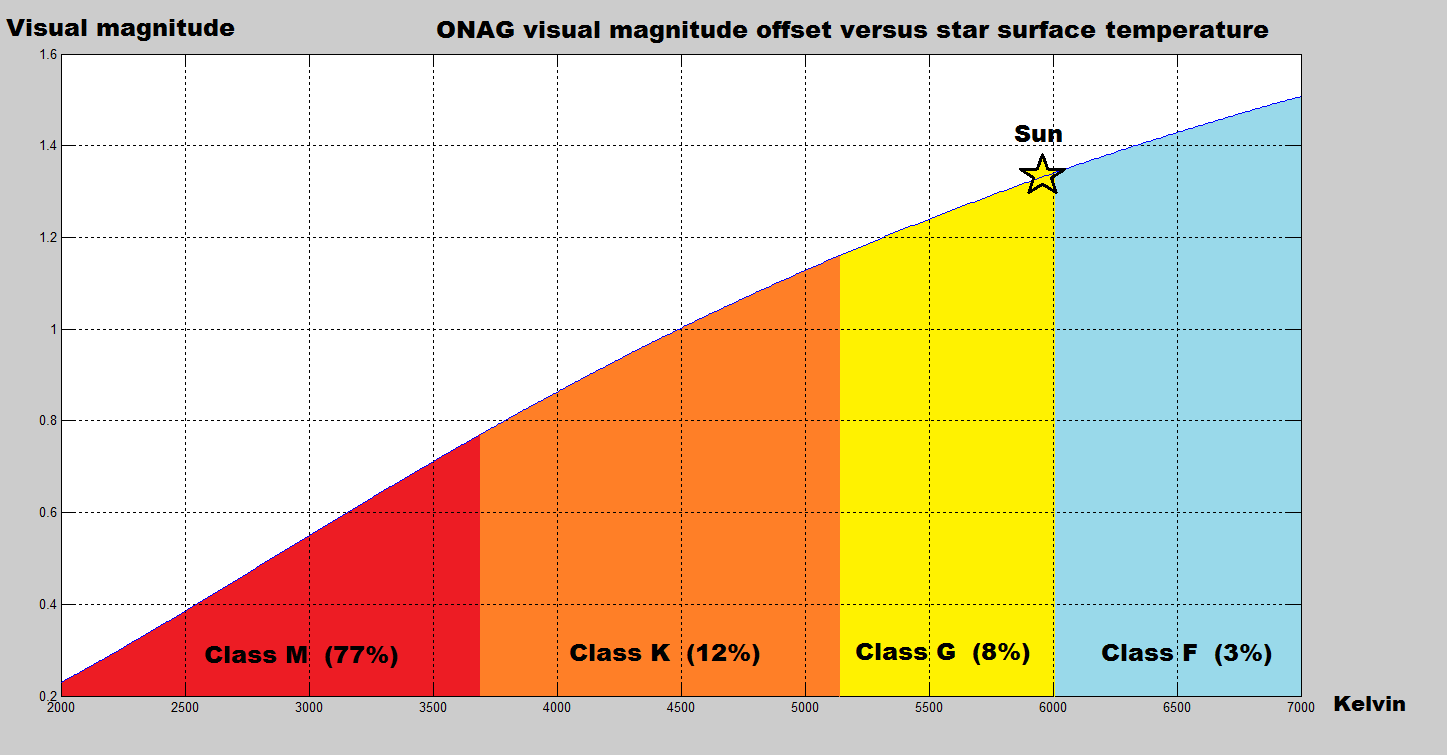
Of course the final level of signal is not only about the star temperature but also the absolute level of energy received here on Earth (luminosity).
Even if a star radiates a lot of NIR how much energy is actually released also matter, as well as the its distance (relative magnitude) to Earth. In actual practical situations one can easily guide with today’s sensor technology at f/10 using a 9th to 10th magnitude and a couple of second exposure in most situations.
Finding a suitable guide star when guiding in the few second range is not a practical limitation or concern.
Also this should be put in perceptive with the ONAG® large field of view (FOV), especially when using its integrated X/Y stage, or guiders with large ships.
The next plot below shows the electromagnetic spectrum from UV to mid infra-read (in microns = 1000nm).
The visible band from 350nm to 750nm is in yellow while the NIR form 750nm to 1000nm (used by the ONAG) is in red. The relative power density spectrum of star with surface temperatures stretching from 3,000K (red) to 7,000K (beginning of the blue) is plotted.
We can see that the black body radiation describing the star spectrum has a quite heavy tail in infrared (IR). When the star surface temperature increases the peak of the spectrum shifts toward the shorter wavelengths, eventually blue hot stars (>7,000k) radiate a lot of energy in the UV band too not accessible for most silicon based sensors (CCD/CMOS) for which the typical QE is limited in the 350nm to 1000nm band.
Star power density spectrum versus surface temperature
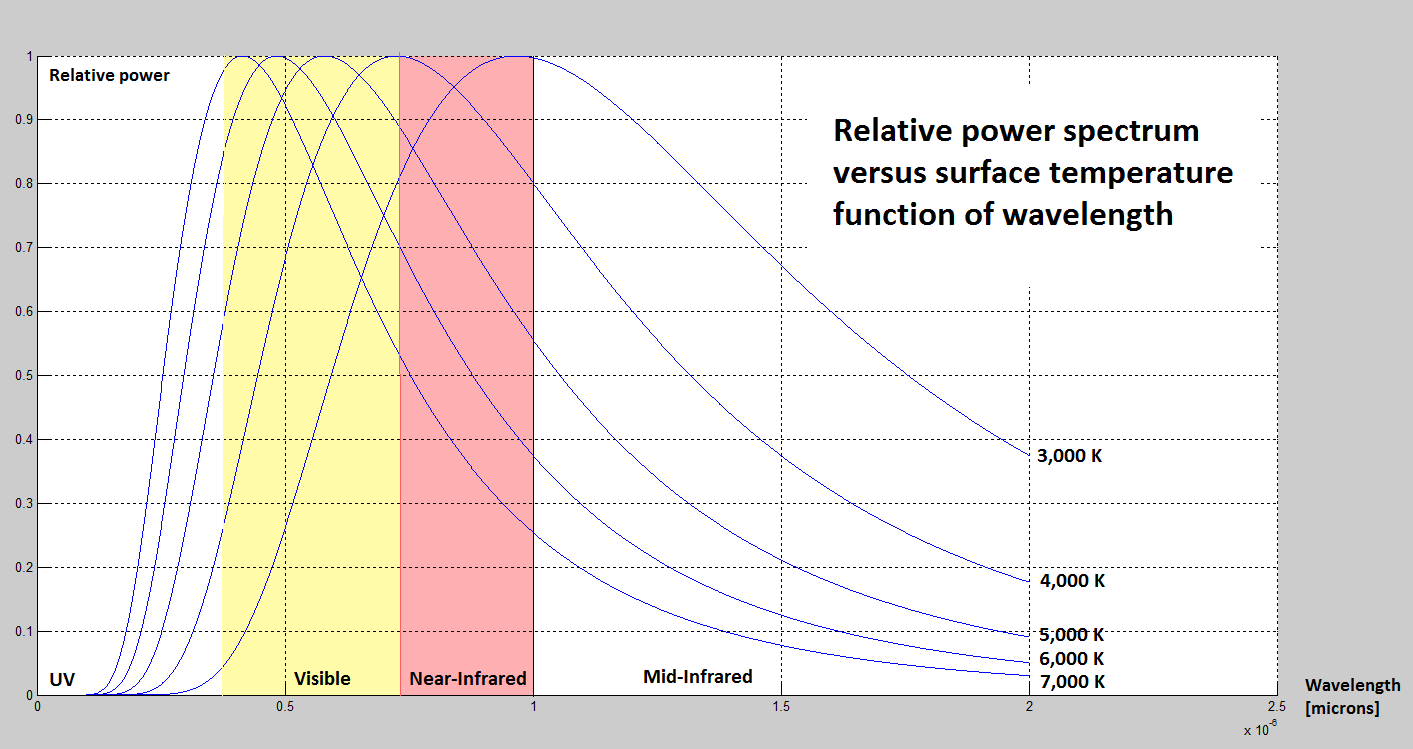
In order to quantify from a practical stand point the impact of using the NIR (750nm to 1000nm) ONAG band for guiding versus the full spectrum accessible to current silicon sensors (visible + NIR), a study was made using the IRSA-PPMXL all sky catalog data (about 900 million objects).
This catalog provides magnitude information for several electromagnetic bands, including the NIR from 750nm to 1000nm. After proper ratiometric calibrations of the visible (B,R) and NIR bands a statistical analysis was perform, the figures below show the ONAG magnitude offset from three different point of views.
ONAG magnitude offset versus star visual magnitudes density plot

All sky ONAG magnitude offset for a star visual magnitude limit of 20

All sky ONAG magnitude offset for a star visual magnitude limit of 10

The signal drop using an ONAG versus the all spectrum stretches typically in average from 0.67 to 1.53 magnitudes, roughly a factor from 2x to 4x.
Depending of the actual silicon sensor QE those average magnitude offsets may need to be adjusted by an extra 0.2 to 0.3 magnitude.
To put this in perspective binning the guide chip by 2×2 already increases at least the SNR by a factor 2 (0.75 magnitude), increasing the guiding exposure time from 1 second to 2.5s seconds (factor 2.5x) increases the guide star limit magnitude by one.
Sketch of OAG/Self Guided camera versus ONAG guiding field of view
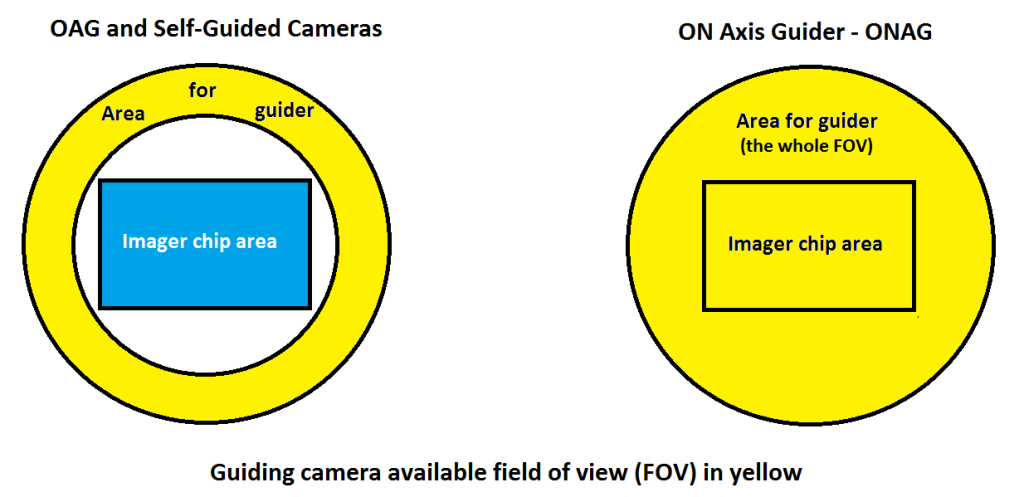
An other important aspect, and key parameter, when auto-guiding is the choice of guider exposure time.
The basic rule is to use the longest exposure one can afford with a given mount and setup (i.e. mount quality, the use of PEC, quality of polar alignment, flexure, the use of point/speed models, …) since we do not want to “chase” the seeing or work with too low SNR values.
One typical upper limit being the inevitable guide star drift, one eventually should keep the guiding exposure such that any drift stays below perception.
With today mounts and a permanent setup it is common to go un-guided for 5 to 10 seconds at least, or even more. In a “grab and go” configuration we are usually in the range of 2 to 5 seconds.
The lower limit on exposure time is then set by the seeing and the SNR of the guide star. Star wander (tilt/tip seeing component) can be significantly reduced (beside using NIR, see below) using few second exposures, for further information on this topic please visit our astronomical seeing education page.
The next plot provides inside on the gain of the guide star limit magnitude when increasing the exposure time relative to one second.
We can see that with ~2.5 second we already gain one magnitude and with ~ 4 seconds we offset the ONAG NIR efficiency with silicon sensors for class F stars. Another good reason to use long enough guiding exposures.
Guide star limit magnitude gain versus exposure time

Reduced seeing effects while guiding in NIR
The seeing will eventually limit the guiding quality. However NIR offers an opportunity to improve the tracking accuracy.
Longer wavelengths are less sensitive to seeing and its effect scales down with the wavelength.
The back and white pictures below of M83 were taken with Dr. M. Motta f/6 – 32″ relay telescope at 14 degrees above the horizon to test seeing effect on guiding in visible (+NIR) or NIR only (>750nm). In both cases the guiding was done using a SBIG AO-L unit. This is obviously an extreme case, which was chosen to challenge auto-guiding under a particular bad seeing condition. The M83 image using full spectrum (vsisible +NIR) experiences even, to some degree, elongated stars. At the time guiding was difficult a best so low above the horizon.
The comparison shows improvement on FWHM and overall image quality using the NIR guiding strategy with an ONAG XT and the adaptive optic AO-L unit. The AO was used not to compensate for the seeing (which it could not do with a so large FOV anyway) but to mitigate the custom mount left over mechanical errors.
The seeing reduction had lower considerably the guide star wander, making auto-guiding tracking much smoother, with less extreme deviations, leading to a better image.
M83 with full spectrum guiding (OAG)
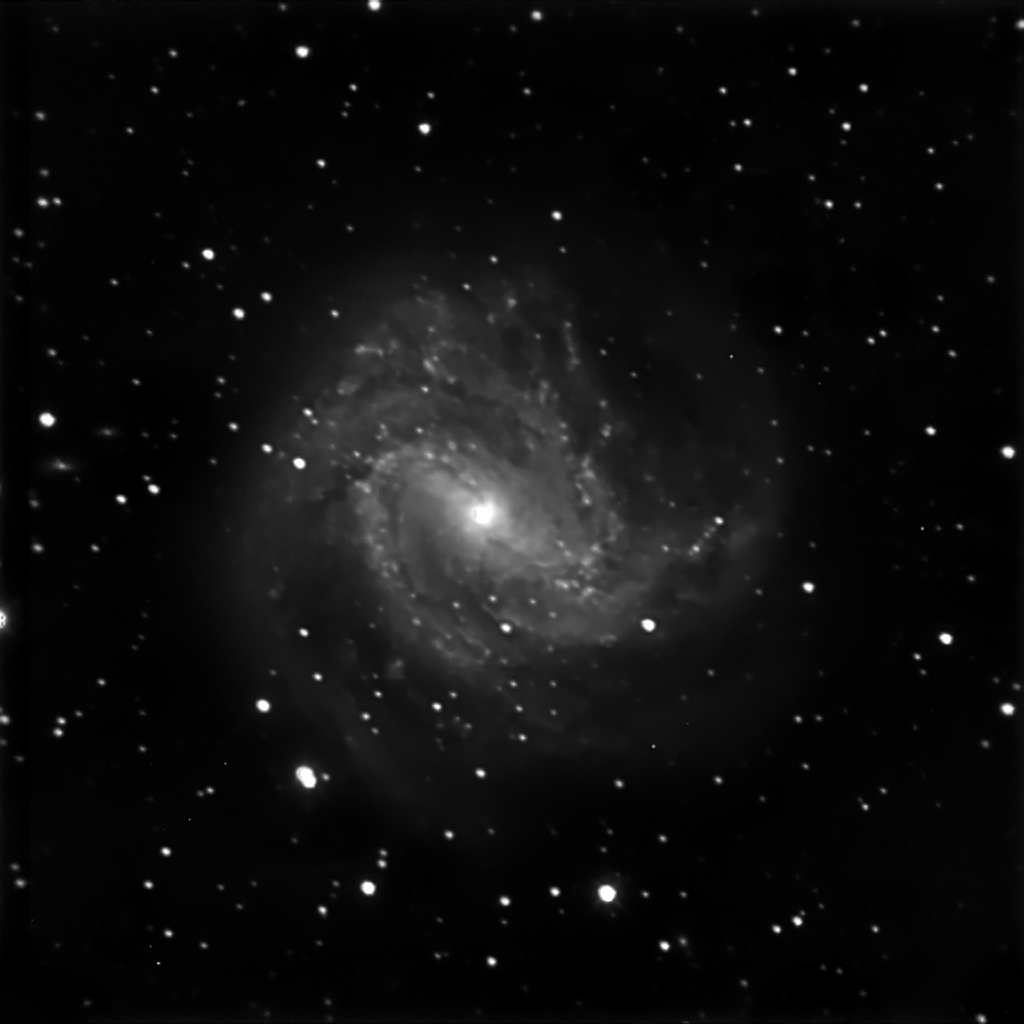
M83 with NIR spectrum guiding (ONAG)
